How the Ocean Floor Helped Seal the Deal for Plate Tectonics
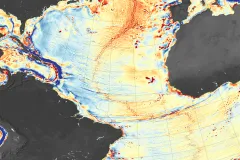
In the early twentieth century, when the Earth scientist Alfred Wegener proposed that the planet’s continents drift — joining to form giant landmasses and retreating away again over long spans of time — most researchers weren’t having it. “Wegener’s hypothesis in general is of the footloose type,” scoffed one geologist, and “takes considerable liberty with our globe.”
That viewpoint was common. Though some scientists accepted Wegener’s proposal, it failed to gain much traction during his lifetime. Much of the Earth science community continued to believe that continents were fixed in place, thanks to a combination of elitism and some genuine scientific weaknesses with Wegener’s theory, like the lack of a plausible explanation for how continents might move across the Earth’s surface.
Today, however, the notion that continents shift their positions over time — though modified since Wegener’s day — is fundamental to the theory of plate tectonics. So, what gives? How did dynamic continents transform from an object of ridicule to a fixture of Geology 101 textbooks?
Much of the answer lies in what might seem an unlikely place: the bottom of the ocean. In the 1950s and 1960s, scientists pieced together several lines of evidence to identify vast, volcanic stretches of the seafloor where lava cools to form Earth’s hard outer crust. It was more than a fascinating find: understanding these areas where crust is born, along with the zones where it dips back into the planet’s interior, allowed scientists to develop the modern theory of plate tectonics and marked a sea change in the field of Earth science.
Earth scientists now see the layered planet as an intensely dynamic system. The Earth’s rigid outer layer, called the lithosphere, is made up of the crust and the shallowest part of the mantle (the shell of rock that lies below the crust). The lithosphere is divided into several rocky puzzle pieces—the tectonic plates—that rest atop the deeper mantle. These plates are constantly shifting, colliding or retreating or sliding past one another, pushed along by the motion of rock in the mantle below.
“This rock, while solid, is also flowing on long timescales,” said Elizabeth Cottrell, a geologist at the Smithsonian National Museum of Natural History. That can be tough to visualize, so Cottrell offers an analogy. “Imagine you tipped a jar of honey on its side in the freezer,” she said. “You could smash the frozen honey with a hammer and shatter it, because on short timescales it behaves like a solid. But on long timescales, the honey would slowly flow and pool in the freezer, behaving like a liquid.”
Earth’s mantle behaves similarly. Even though it’s solid, it flows very slowly, with warmer, deeper rock rising toward the surface before cooling and sinking back down. This process, known as convection, acts like a conveyor belt for the lithosphere above it. As rock convects in the mantle, it shuttles the tectonic plates around the Earth’s surface.
But even as recently as the mid-twentieth century, most scientists thought of the Earth as a far more static place. Many assumed that the seafloor, which we now know is rugged, active, and ever-changing, was like the bottom of a planetary bathtub, flat and featureless and ultimately uninteresting.
That assumption began to crumble in the 1950s, thanks in large part to the pioneering work of the oceanographic cartographer Marie Tharp. Using sonar data from ships that had entered the ocean in droves during World War II, Tharp mapped the topography of a seafloor that looked nothing like the bottom of a bathtub. Instead, it was a landscape as complex as the terrestrial one, complete with towering mountains, yawning trenches, and sprawling plains. One of Tharp’s most stunning finds was the Mid-Atlantic Ridge, a gigantic mountain range that spans the Atlantic Ocean. We now know that this ridge marks the boundary where multiple pairs of tectonic plates pull away from one another at a few centimeters per year.
Tharp’s colleagues ridiculed some of her work, including her hypothesis that new crust formed at sites like the Mid-Atlantic Ridge. But other scientists were coming to similar conclusions. In 1962, for instance, the geologist Harry Hess formally proposed the concept that would become known as seafloor spreading. At plate boundaries like the Mid-Atlantic Ridge, he thought, molten rock from deep inside the Earth would upwell to the surface and cool, producing new oceanic crust.
Today, Earth scientists accept seafloor spreading as an integral part of plate tectonics. “When you have two oceanic plates moving away from one another, that’s called a spreading center,” said Daniel Rasmussen, a volcanologist and Peter Buck postdoctoral fellow at the museum. As mantle rock convects upward, he said, the decreasing pressure will cause it to melt. “And then that melt will percolate up into the spreading center, and some of it will erupt at the surface.” Bathed by ocean water, the lava cools, and you have new seafloor.
That doesn’t mean the planet is growing. “The crust is being consumed and recycled back into Earth’s interior at an equal rate, at subduction zones,” Cottrell said. At these zones, the boundaries where tectonic plates collide into each other, one plate slides beneath the other. As the descending plate churns and releases its water, it causes surrounding rock to melt into magma, meaning subduction zones are sites of intense volcanic activity.
None of these concepts had been solidified when scientists like Tharp and Hess were investigating the seafloor. Instead, the notion that the seafloor was full of ruptures, pulling apart and birthing new crust like so many planetary nurseries, was radical. It wasn’t lost on the Earth science community that this process would provide a basis for the much-maligned theory of continental drift, since continental crust would move just like oceanic crust. Such a blow to the prevailing model would require some hefty evidence.
It didn’t take long to arrive. Some of the most compelling evidence came in the 1960s, from distinctive patterns found in magnetic minerals embedded in the rock at deep-sea spreading centers.
When magnetic minerals in lava cool down, they orient themselves according to Earth’s magnetic field, like tiny compasses. Because the direction of Earth’s magnetic field has flipped several times over the planet’s history, the orientation of the minerals differs depending on when they formed. That means spreading centers, where new minerals are constantly forming, should act like a logbook of the planet’s magnetic history. Sure enough, these sites show symmetrical patterns of magnetic “stripes,” as new minerals repeatedly lock in the direction of the field and spread outward.
The magnetic stripe observations, combined with other lines of evidence — including volcanic activity, earthquakes, and data that Wegener had marshaled in support of his theory decades previously — started to convince scientists of the Earth’s dynamic structure.
“There were all these ideas around that were consistent with plate tectonics, and the theory tied them all together,” Rasmussen said. “Gaining an understanding of how seafloor is formed helped scientists understand what they had been seeing on land surfaces for many, many years.”
Spreading centers like the Mid-Atlantic Ridge are a crucial part of the Earth system for a number of reasons. Their volcanism produces energy that supports vibrant deep-sea communities. They are sites of seawater circulation, playing a vital role in ocean chemistry. And they’ve been key players in the history of Earth science, helping scientists understand the workings of a planet in motion.